INTERFERin® provides very high silencing efficiency already at 1 nM siRNA and can be used in a wide variety of adherent and suspension cells....
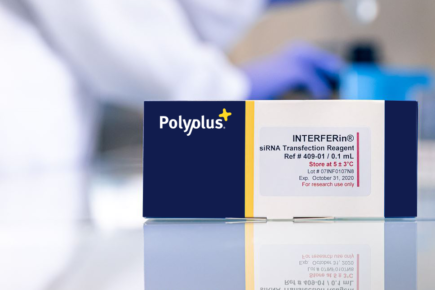
We use cookies to help you navigate efficiently and perform certain functions. You will find detailed information about all cookies under each consent category below.
The cookies that are categorized as "Necessary" are stored on your browser as they are essential for enabling the basic functionalities of the site. ...
Necessary cookies are required to enable the basic features of this site, such as providing secure log-in or adjusting your consent preferences. These cookies do not store any personally identifiable data.
Functional cookies help perform certain functionalities like sharing the content of the website on social media platforms, collecting feedback, and other third-party features.
Analytical cookies are used to understand how visitors interact with the website. These cookies help provide information on metrics such as the number of visitors, bounce rate, traffic source, etc.
Advertisement cookies are used to provide visitors with customized advertisements based on the pages you visited previously and to analyze the effectiveness of the ad campaigns.
The delivery of RNAi machinery is a powerful tool to explore treatment options for many diseases and also in furthering our understanding of mechanistic interplay in cellular signalling pathways and functionality. Here, as shown through multiple recently publish examples, INTERFERin® and in vivo jetPEI® have a proven track record of highly effective RNAi delivery both in vitro and in vivo and thus are competitive and reliable choices for future genetic and biological research
RNAi refers to the phenomenon of RNA interference and was first discovered in 1990 where scientists observed that particular RNA strands had the ability to suppress gene expression in plants (1). This discovery catalysed a plethora of research focused on unpicking the RNAi mechanism of action and about 10 years later it was established that RNAi results in a sequence specific manner(2), and can be achieved using the following examples:
More specifically, RNAi mechanism sees these small pieces of RNA block post-transcriptional translation and thus silencing protein production for particular genes/sets of genes. This is a completely natural process in normal cell regulation but opens up vast opportunities for gene therapy applications for the treatment or management of genetic disorders such as cancer or muscular dystrophy for example. Of course, any gene therapy is only as good as it’s delivery system, hence in this article we shall discuss the process of transfection and highlight two highly effective vectors for both in vitro and in vivo gene therapy applications.
Firstly, it is important to understand the concept of transfection. Transfection involves the delivery of nucleic acids into cells in order to modify their transcriptional repertoire. However, this is not a straight foreword process as nucleic acids (i.e., RNA, DNA, microRNA, siRNA etc) as well as cell membrane both have a net negative charge, thus naturally repel one another. In order to overcome this barrier positively charged transfection reagents can be used to package genetic cargo and deliver it across cell membranes. These positively charged polymers are electrostatically attracted to the negatively charged nucleic acids and are able to complex into cationic nanoparticles via electrostatic interaction, giving them the ideal biophysical characteristics to enter in cells. This form of chemical mediated transfection is advantageous as it is fast, simple to use and reproducible. Polyplus–transfection offers world-class transfection reagents that can be used for effective and reproducible delivery of RNAi machinery both in vitro in a wide variety of cell types and in vivo, and so let us discuss in more details two of our top reagents: INTERFERin® and in vivo–jetPEI®.
Schematic of siRNA transfection with INTERFERin® for transient gene silencing
INTERFERin® is a transfection reagent utilized for the delivery of siRNA and microRNA. The advantage of using INTERFERin® is that it can delivery low concentrations of siRNA which helps circumvent off-target gene inhibition in cultured cells. Thus, INTERFERin® yields highly reproducible experimental outcomes. With these attributes INTERFERin® has an edge on other leading transfection reagents, and this is evidenced by its frequent use in high-impact publications, with more than 145 high quality publications since 2019 using this product for research not only exploring mechanistic actions of RNAi on cellular response and signalling, but also on various diseases including fibrosis, cancer, and obesity.
For example, in a paper published by Bisi et al., (Nature Communications, 2020) (3), the authors used INTERFERin® to study how the IRSp53 gene controls plasma membrane shape and polarised transport within epithelial tubules. INTERFERin® was used here to transfect MDCK cells with an IRSp53 targeting siRNA for transient RNAi interference experiments. Through these studies it was shown that genetic removal of IRSp53 lead to abnormalities in renal tubulogenesis. A further example comes from Kunz et al., (Journal of Nanobiotechnology, 2020)(4), in which INTERFERin® was used in experiments delivering antimiRs as therapeutics for both prostate carcinoma and melanoma cells. Here, the authors used INTERFERin® to create nanocomplexes with antimiRs for transfection studies, achieving significant knockdown in vitro. These studies highlighted and explored the therapeutic relevance of microRNAs-375,-141,-150, and-638 as targets for oncogenic activity.
The translation of in vitro work into pre-clinical models is paramount for advancing new therapeutics into the clinic. For in vivo delivery of RNAi reagents, Polyplus–transfection offers an excellent product called in vivo-jetPEI®. This product has shown efficacy at in vivo delivery not only of DNA, but also siRNA, microRNA and shRNA. in vivo-jetPEI® has shown efficiency across a range of animal models including the use of mice, rats, rabbit, dog and monkeys, as well as potent effects via a range of administration route, including local and systemic injection. With in vivo-jetPEI® being easy to use and versatile, it allows for highly translatable research in vivo, and this is manifested in its use across many disease models and biomedical research disciplines, with more than 90 publications using this product in high–impact journals since 2019. Research disciplines include neurological diseases, diabetes, and cancer to name a few.
For example, Tanaka et al.,(Nature Communications, 2020)(5) used in vivo-jetPEI® for siRNA mediated knockdown of yes-associated protein 1 (YAP) in a mouse model of Alzheimer’s disease via direct injection into the retrosplenial cortex. This research revealed that YAP signalling leads to necrosis in early-stage Alzheimer’s disease and so opens up avenues to explore future treatments for the disease.
Moreover, Su et al., (Molecular Cell, 2019)(6) explored the mechanistic interactions of Heat shock factor 1 and AMP-Activated protein Kinase. In this study, the authors utilized in vivo-jetPEI® to deliver shRNAs in a range of mouse genotypes via tail vein injection. This work discovered a new layer of regulation between heat shock factor 1 and AMP-Activated protein Kinase revealing that they constitute a mutually repressive complex. With Heat shock factor 1 governing the biological response to proteotoxic stress, as well as being identified as a pro–oncogenic, this research will help further understand the mechanisms behind these processes and potential reveal new therapeutic targets.
References